iNANO Scientists Publish in Physical Review Letters
iNANO Scientists Solve an Important Oxide Surface Structure for Heterogeneous Catalysis
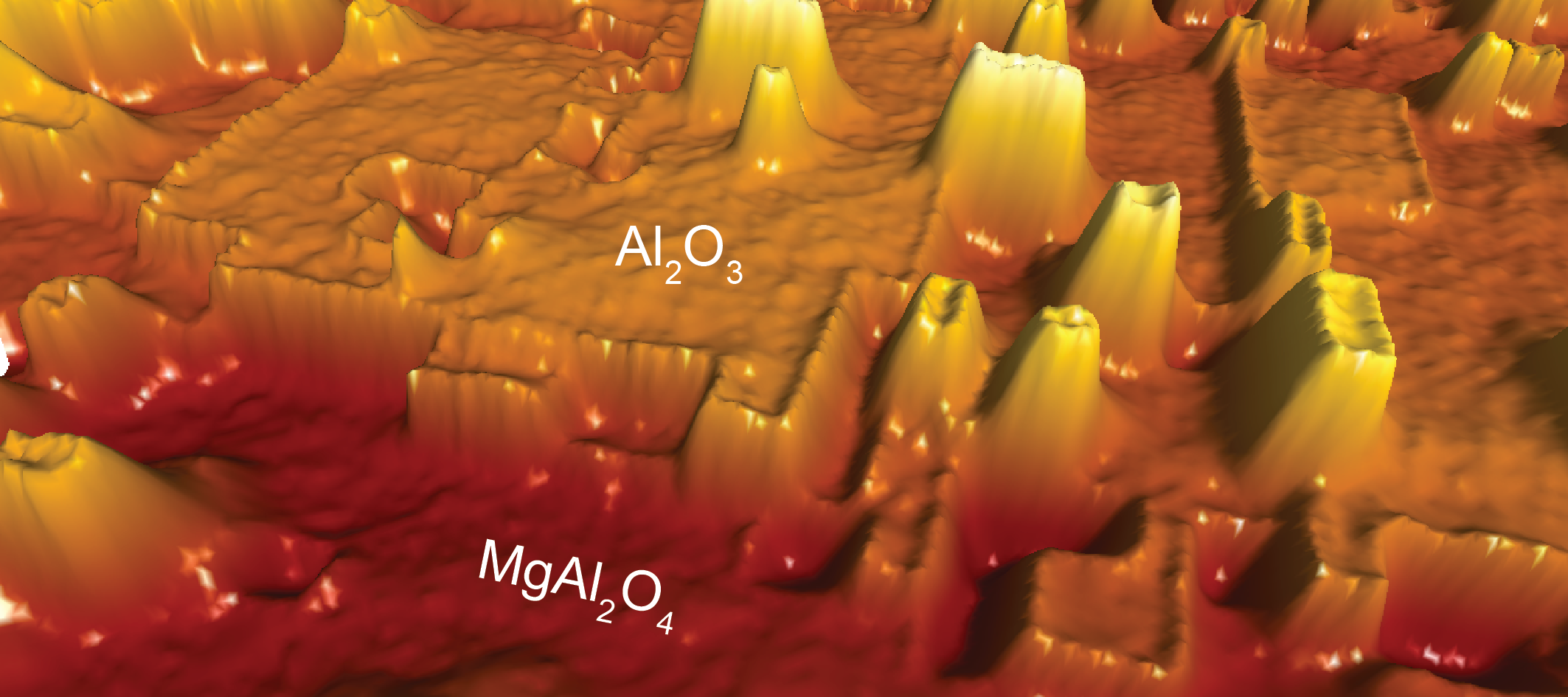
Heterogeneous catalysis plays a crucial role in today’s modern society. It protects the environment, for example by cleaning up the exhaust gas from cars, and it serves as the backbone technology for the production of almost all chemicals. In order to develop new and better catalysts, it is important to understand the detailed structure of catalyst materials, but to this day, the structure of some of the most important catalyst materials remain unknown. Now, iNANO scientist have gained new insight into the structure of one of the most widely used oxide materials in catalysis.
Catalysts and Support Materials
A catalyst speeds up a chemical reaction many times compared to the non-catalytic reaction, and most importantly, the catalyst remains unchanged and can be used over and over to catalyse the same reaction. Catalysts thereby make it industrially feasible to produce very large amounts of chemicals every single day. You can say that they are the workhorses in the chemical industry, where around 90 % of the products of are made in catalytic processes. In heterogeneous catalysis, the catalyst is in solid form and catalyse reactions of molecules in solution or gas. Often, catalysts are scarce and very expensive (e.g. platinum) and for that reason they are usually extremely small nanometer-sized particles supported on a much cheaper support material. The support material has to be able to bind strongly to the catalyst particles in order to prevent that they melt together and form big particles. This process is called sintering and it is the most common cause of catalyst deactivation. To develop new and better catalysts we need to fundamentally understand the surface structure of the catalyst support materials and also the interaction between the catalyst particles and the support materials.
Studying the Perfect Support Material
The most widely used support material is alumina (Al2O3), because it is cheap and it remains stable up to very high temperatures. The transition aluminas are especially interesting because they have extremely large surface areas, so they can support a huge amount of catalyst particles per volume, thereby increasing the catalytic activity. Unfortunately, these transition aluminas are difficult to study for two reasons: firstly, because they are non-conductive, only a very limited amount of experimental techniques can be used, and secondly, it is impossible to grow large crystals of these materials, thereby making it impossible to study the surface structure.
To circumvent the first problem, we use a state of the art microscope, a so-called non-contact atomic force microscope. The biggest strength of this microscope is that we can study both conducting materials like metals but also non-conducting metal oxides like alumina, and these materials can be studied with atomic resolution.
The second problem is a bit more tricky to overcome. Here we need to take advantage of a trick called epitaxial growth, where we can stimulate a material to adapt a desired structure. In our case we slowly grow ultra-thin transition alumina films on magnesium aluminate (MgAl2O4) substrates, and by using this method, we can trick the system into thinking it is γ-Al2O3.
Success Thanks to International Collaborations
The transition alumina films have been primarily studied with our atomic force microscope, and obtaining an even better understanding of the structure has only been possibly through fruitful international and interdisciplinary collaborations with research groups in the United States, Germany and Finland. At Yale University our samples have been studied with a technique called low-energy electron diffraction, and together with researchers from the University of Hamburg, we went to the European Synchrotron Radiation Facility in Grenoble, France, and obtained some spectacular results. In Helsinki, a research group has performed numerous theoretical calculations on huge supercomputers to support all of our experimental findings, and finally, researches at Haldor Topsøe A/S have also contributed to solving the exact structure of the transition alumina films.
For more information contact:
Thomas Nørregaard Jensen
PhD Student, iNANO, Aarhus University
tnj@inano.au.dk
or Jeppe Vang Lauritsen
Associate Professor, iNANO, Aarhus University
jvang@inano.au.dk
References
- I. Chorkendorff and J. W. Niemantsverdriet, Concepts of Modern Catalysis and Kinetics (Wiley-VCH Verlag, Weinheim, 2007), 2nd edn.